Terra Sirenum
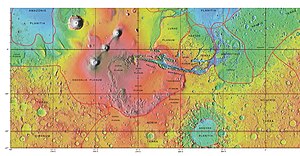

Terra Sirenum is a large region in the southern hemisphere of the planet Mars. It is centered at 39°42′S 150°00′W / 39.7°S 150°W and covers 3900 km at its broadest extent. It covers latitudes 10 to 70 South and longitudes 110 to 180 W.[1] Terra Sirenum is an upland area notable for massive cratering including the large Newton Crater. Terra Sirenum is in the Phaethontis quadrangle and the Memnonia quadrangle of Mars. A low area in Terra Sirenum is believed to have once held a lake that eventually drained through Ma'adim Vallis.[2][3][4]
Terra Sirenum is named after the Sirens, who were birds with the heads of girls. In the Odyssey these girls captured passing seamen and killed them.[5]
Chloride deposits
Evidence of deposits of chloride based minerals in Terra Sirenum was discovered by the 2001 Mars Odyssey orbiter's Thermal Emission Imaging System in March 2008. The deposits are approximately 3.5 to 3.9 billion years old. This suggests that near-surface water was widespread in early Martian history, which has implications for the possible existence of Martian life.[6][7] Besides finding chlorides, MRO discovered iron/magnesium smectites which are formed from long exposure in water.[8]
Based on chloride deposits and hydrated phyllosilicates, Alfonso Davila and others believe there is an ancient lakebed in Terra Sirenum that had an area of 30,000 km2 and was 200 meters deep. Other evidence that supports this lake are normal and inverted channels like ones found in the Atacama desert.[9]
Inverted relief
Some areas of Mars show inverted relief, where features that were once depressions, like streams, are now above the surface. It is believed that materials like large rocks were deposited in low-lying areas. Later, erosion (perhaps wind which can't move large rocks) removed much of the surface layers, but left behind the more resistant deposits. Other ways of making inverted relief might be lava flowing down a stream bed or materials being cemented by minerals dissolved in water. On Earth, materials cemented by silica are highly resistant to all kinds of erosional forces. Examples of inverted channels on Earth are found in the Cedar Mountain Formation near Green River, Utah. Inverted relief in the shape of streams are further evidence of water flowing on the Martian surface in past times.[10]
-
CTX image of craters with black box showing location of next image.
-
Image from previous photo of a curved ridge that may be an old stream that has become inverted. Image taken with HiRISE under the HiWish program.
Martian gullies
Terra Sirenum is the location of many Martian gullies that may be due to recent flowing water. Some are found in the Gorgonum Chaos[11][12] and in many craters near the large craters Copernicus and Newton.[13][14] Gullies occur on steep slopes, especially on the walls of craters. Gullies are believed to be relatively young because they have few, if any craters. Moreover, they lie on top of sand dunes which themselves are considered to be quite young.
-
CTX image of the next image showing a wide view of the area. Since the hill is isolated it would be difficult for an aquifer to develop. Rectangle shows the approximate location of the next image.
-
Gully on mound as seen by Mars Global Surveyor, under the Public Target Program. Images of gullies on isolated peaks, like this one, are difficult to explain with the theory of water coming from aquifers because aquifers need large collecting areas.
-
Another view of the previous gully on a mound. This one is with HiRISE, under the HiWish program. This view shows most of the apron and two old glaciers associated with it. All that is left of the glaciers are terminal moraines.
-
MOLA context image for the series of three images to follow of gullies in a trough and nearby crater.
-
Gullies in a trough and nearby crater, as seen by HiRISE under the HiWish program. Scale bar is 500 meters long.
-
Close-up of gullies in crater, as seen by HiRISE under the HiWish program.
-
Close-up of gullies in trough, as seen by HiRISE under the HiWish program. These are some of the smaller gullies visible on Mars.
-
HiRISE image, taken under HiWish program, of gullies in a crater in Terra Sirenum.
-
Gullies with remaines of a former glacier in crater in Terra Sirenum, as seen by HiRISE under HiWish program.
-
Gullies in a crater in Terra Sirenum, as seen by HiRISE under the HiWish Program.
-
Close-up of gully showing multiple channels and patterned ground, as seen by HiRISE under the HiWish program.
-
Gullies in crater in Phaethontis quadrangle, as seen by HiRISE under HiWish program
-
Gullies in crater, as seen by HiRISE under HiWish program. Location is Phaethontis quadrangle.
-
Close up of gullies in crater showing channels within larger valleys and curves in channels. These characteristics suggest they were made by flowing water. Note: this is an enlargement of the previous image by HiRISE under HiWish program. Location is Phaethontis quadrangle.
-
Close up of gully network showing branched channels and curves; these characteristics suggest creation by a fluid. Note: this is an enlargement of a previous wide view of gullies in a crater, as seen by HiRISE under HiWish program. Location is Phaethontis quadrangle.
-
Gullies in two levels of a crater wall, as seen by HiRISE under HiWish program. Gullies at two levels suggests they were not made with an aquifer, as was first suggested. Location is Phaethontis quadrangle.
-
Image of gullies with main parts labeled. The main parts of a Martian gully are alcove, channel, and apron. Since there are no craters on this gully, it is thought to be rather young. Picture was taken by HiRISE under HiWish program. Location is Phaethontis quadrangle.
-
Close-up of gully aprons showing they are free of craters; hence very young. Location is Phaethontis quadrangle. Picture was taken by HiRISE under HiWish program.
Tongue-shaped glaciers
-
Tongue-shaped glacier, as seen by HiRISE under the HiWish program. Location is Phaethontis quadrangle.
-
Wide view of several tongue-shaped glaciers on wall of crater, as seen by HiRISE under the HiWish program. The glaciers are of different sizes and lie at different levels. Some of these are greatly enlarged in pictures which follow.
-
Close-up of the snouts of two glaciers from the previous image, as seen by HiRISE under the HiWish program. These are towards the bottom left of the previous image.
-
Close-up of small glaciers from a previous image, as seen by HiRISE under the HiWish program. Some of these glaciers seem to be just starting to form.
-
Close-up of the edge of one of the glaciers on the bottom of the wide view from a previous image Picture was taken by HiRISE under the HiWish program.
Possible pingos
The radial and concentric cracks visible here are common when forces penetrate a brittle layer, such as a rock thrown through a glass window. These particular fractures were probably created by something emerging from below the brittle Martian surface. Ice may have accumulated under the surface in a lens shape; thus making these cracked mounds. Ice being less dense than rock, pushed upwards on the surface and generated these spider web-like patterns. A similar process creates similar sized mounds in arctic tundra on Earth. Such features are called “pingos,”, an Inuit word.[15] Pingos would contain pure water ice; thus they could be sources of water for future colonists of Mars.
-
Possible pingo, as seen by HiRISE under HiWish program
-
Possible pingos with scale, as seen by HiRISE under HiWish program
-
Close view of possible pingo with scale, as seen by HiRISE under HiWish program
-
Example of a pingo on Earth. On Earth the ice that caused the pingo would melt and fill the fractures with water; on Mars the ice would turn into a gas in the thin Martian atmosphere.
Concentric crater fill
Concentric crater fill, like lobate debris aprons and lineated valley fill, is believed to be ice-rich.[16] Based on accurate topography measures of height at different points in these craters and calculations of how deep the craters should be based on their diameters, it is thought that the craters are 80% filled with mostly ice.[17][18][19][20] That is, they hold hundreds of meters of material that probably consists of ice with a few tens of meters of surface debris.[21][22] The ice accumulated in the crater from snowfall in previous climates.[23][24][25] Recent modeling suggests that concentric crater fill develops over many cycles in which snow is deposited, then moves into the crater. Once inside the crater shade and dust preserve the snow. The snow changes to ice. The many concentric lines are created by the many cycles of snow accumulation. Generally snow accumulates whenever the axial tilt reaches 35 degrees.[26]
-
Crater showing concentric crater fill, as seen by CTX (on Mars Reconnaissance Orbiter). Location is Phaethontis quadrangle.
-
Close-up view of concentric crater fill, as seen by HiRISE under HiWish program Note: this is an enlargement of previous image of a concentric crater. Location is Phaethontis quadrangle.
-
Wide view of concentric crater fill, as seen by CTX Location is the Phaethontis quadrangle.
-
Concentric crater fill, as seen by HiRISE under HiWish program Location is the Phaethontis quadrangle.
-
Close, color view of concentric crater fill, as seen by HiRISE under HiWish program Location is the Phaethontis quadrangle.
Liu Hsin Crater features
-
Liu Hsin Crater, as seen by CTX camera (on Mars Reconnaissance Orbiter).
-
Dunes in Liu Hsin Crater, as seen by CTX camera (on Mars Reconnaissance Orbiter). Dark lines are dust devil tracks. Note: this is an enlargement of a previous image of Liu Sin Crater.
-
Dust devil tracks in Liu Hsin Crater, as seen by CTX camera (on Mars Reconnaissance Orbiter). Gullies can also be seen on the crater wall, near the bottom of picture. Note: this is an enlargement of a previous image of Liu Sin Crater.
-
Gullies in Liu Hsin Crater, as seen by HiRISE under HiWish program. Curved lines on crater floor may be remains of old glaciers.
Magnetic stripes and plate tectonics
The Mars Global Surveyor (MGS) discovered magnetic stripes in the crust of Mars, especially in the Phaethontis and Eridania quadrangles (Terra Cimmeria and Terra Sirenum).[27][28] The magnetometer on MGS discovered 100 km wide stripes of magnetized crust running roughly parallel for up to 2000 km. These stripes alternate in polarity with the north magnetic pole of one pointing up from the surface and the north magnetic pole of the next pointing down.[29] When similar stripes were discovered on Earth in the 1960s, they were taken as evidence of plate tectonics. Researchers believe these magnetic stripes on Mars are evidence for a short, early period of plate tectonic activity. When the rocks became solid they retained the magnetism that existed at the time. A magnetic field of a planet is believed to be caused by fluid motions under the surface.[30][31][32] However, there are some differences, between the magnetic stripes on Earth and those on Mars. The Martian stripes are wider, much more strongly magnetized, and do not appear to spread out from a middle crustal spreading zone. Because the area containing the magnetic stripes is about 4 billion years old, it is believed that the global magnetic field probably lasted for only the first few hundred million years of Mars' life, when the temperature of the molten iron in the planet's core might have been high enough to mix it into a magnetic dynamo. There are no magnetic fields near large impact basins like Hellas. The shock of the impact may have erased the remnant magnetization in the rock. So, magnetism produced by early fluid motion in the core would not have existed after the impacts.[33]
When molten rock containing magnetic material, such as hematite (Fe2O3), cools and solidifies in the presence of a magnetic field, it becomes magnetized and takes on the polarity of the background field. This magnetism is lost only if the rock is subsequently heated above a particular temperature (the Curie point which is 770 °C for iron). The magnetism left in rocks is a record of the magnetic field when the rock solidified.[34]
Other features
-
Channel, as seen by HiRISE under HiWish program Streamlined shapes are indicated with arrows. Location is the Phaethontis quadrangle.
-
Possible chloride deposits in Terra Sirenum
-
Layers in crater wall, as seen by HiRISE under HiWish program. Area in box is enlarged in the next image.
-
Enlargement from previous image, showing many thin layers. Note that the layers do not seem to be formed from rocks. They may be all that is left of a deposit that once filled the crater. Image was taken with HiRISE, under HiWish program.
-
Surface of crater floor, as seen by HiRISE under HiWish program.
-
Surface of crater floor showing details from image taken with HiRISE, under HiWish program. This may be a transition from one type of structure to a different, maybe due to erosion.
-
Surface showing large hollows of unknown origin, as seen by HiRISE under HiWish program. The hollows may be the result of large amounts of ice leaving the ground.
-
Close-up of surface with large hollows, as seen by HiRISE under HiWish program.
-
Layers in mantle, as seen by HiRISE under HiWish program.
-
Oxbow lake, as seen by HiRISE under HiWish program.
-
Troughs on the floor of Bernard Crater showing many boulders, as seen by HiRISE under HiWish program
-
Troughs on the floor of Bernard Crater, as seen by HiRISE under HiWish program
-
Large pits in Sirenum Fossae, as seen by HiRISE under HiWish program
-
Lava flow. Lava flow stopped when it encountered the higher ground of a mound. Picture was taken with HiRISE under HiWish program.
-
HiRISE image showing smooth mantle covering parts of a crater in the Phaethontis quadrangle. Along the outer rim of the crater, the mantle is displayed as layers. This suggests that the mantle was deposited multiple times in the past. Picture was taken with HiRISE under HiWish program. The layers are enlarged in the next image.
-
Enlargement of previous image of mantle layers. Four to five layers are visible. Location is the Phaethontis quadrangle.
-
Surface showing appearance with and without mantle covering, as seen by HiRISE, under the HiWish program. Location is Terra Sirenum in Phaethontis quadrangle.
Interactive Mars map
<imagemap>
Image:Mars Map.JPG|thumb|center|620px|alt=Map of Mars|
poly 377 357 423 380 407 405 369 389 Acheron Fossae poly 1316 75 1305 231 992 408 677 224 651 71 1069 169 Acidalia Planitia poly 564 320 555 402 464 416 449 348 489 317 Alba Mons poly 160 408 355 401 311 594 156 594 Amazonis Planitia poly 832 741 903 744 897 793 749 880 776 950 831 1052 829 1121 510 1126 653 854 Aonia Planitia poly 1186 320 1477 394 1040 732 960 635 952 525 Arabia Terra poly 155 70 578 72 580 258 156 429 377 238 284 224 308 283 158 408 Arcadia Planitia poly 875 1108 872 1128 956 1131 957 1041 906 1047 904 1084 Argentea Planum poly 871 871 935 887 926 942 884 967 837 918 Argyre Planitia poly 880 356 962 441 889 505 824 412 Chryse Planitia poly 515 777 575 751 671 792 684 835 580 854 Claritas Fossae poly 981 456 992 493 1076 412 1044 386 Cydonia Mensae poly 346 685 514 740 526 838 363 797 Daedalia Planum poly 1858 405 1968 412 1954 514 1860 505 Elysium Mons poly 1692 500 1945 509 2009 342 2092 588 2085 671 2007 689 Elysium Planitia poly 1848 617 1870 616 1882 638 1878 637 1848 639 Gale crater poly 1645 865 1675 858 1645 782 1600 789 Hadriaca Patera poly 1378 766 1418 783 1392 882 1356 874 Hellas Montes poly 1410 772 1515 769 1618 869 1493 943 1387 894 Hellas Planitia poly 1599 731 1610 599 1711 593 1750 717 Hesperia Planum poly 928 703 919 746 960 761 959 726 Holden crater poly 524 827 588 867 564 900 511 881 Icaria Planum poly 1587 447 1672 520 1619 575 1555 568 1538 500 Isidis Planitia poly 1531 475 1518 514 1547 516 1552 484 Jezero crater poly 1032 72 1030 87 1112 94 1124 166 1032 170 Lomonosov crater poly 153 569 280 579 293 650 156 651 Lucus Planum poly 356 411 400 451 357 486 329 465 Lycus Sulci poly 1243 254 1311 258 1309 310 1244 304 Lyot crater poly 738 489 823 493 792 597 704 573 Lunae Planum poly 1367 964 1552 989 1531 1099 1361 1078 Malea Planum poly 832 1047 920 1048 916 1124 839 1125 Maraldi crater poly 572 326 612 356 676 303 658 276 Mareotis Fossae poly 664 301 752 369 700 454 628 400 Mareotis Tempe poly 872 491 976 477 1054 609 953 635 Margaritifer Terra poly 1853 283 1857 333 1903 330 1899 281 Mie crater poly 309 233 346 235 344 265 303 267 Milankovič crater poly 1694 508 1665 562 1767 637 1798 575 Nepenthes Mensae poly 801 855 931 819 941 861 871 857 848 883 Nereidum Montes poly 1529 438 1446 412 1462 371 1525 404 Nilosyrtis Mensae poly 1197 672 994 834 978 1072 1276 1086 1365 785 Noachis Terra poly 471 410 547 418 532 488 479 470 Olympica Fossae poly 390 417 481 505 373 547 353 483 Olympus Mons poly 137 1061 139 1144 2102 1143 2103 1063 Planum Australe poly 1804 1124 1844 649 1663 650 1592 1129 Promethei Terra poly 1276 219 1262 253 1373 329 1383 302 Protonilus Mensae poly 151 729 314 737 323 852 156 885 Sirenum poly 1370 899 1432 952 1373 972 1339 933 Sisyphi Planum poly 585 631 784 692 697 823 574 807 Solis Planum poly 570 638 608 673 574 707 531 670 Syria Planum poly 602 232 645 243 595 314 561 306 Tantalus Fossae poly 727 306 790 260 840 392 731 497 693 567 Tempe Terra poly 1850 652 2080 675 2086 1128 1799 1125 Terra Cimmeria poly 1403 483 1547 596 1476 690 1350 749 1242 696 1214 642 Terra Sabaea poly 158 816 589 900 655 1124 171 1123 Terra Sirenum poly 547 484 611 530 488 714 413 656 Tharsis Montes poly 576 385 604 401 585 445 564 428 Tractus Catena poly 1583 607 1782 679 1762 800 1604 771 1531 738 Tyrrhena Terra poly 452 529 505 568 475 583 445 570 Ulysses Patera poly 608 432 641 439 633 467 605 456 Uranius Patera poly 1413 79 1421 296 1627 447 1820 471 1853 364 1832 277 1894 254 1918 311 1829 476 2079 214 2078 80 Utopia Planitia poly 589 582 907 651 865 716 572 655 Valles Marineris poly 139 51 142 132 2103 133 2104 53 Vastitas Borealis poly 824 475 915 593 889 632 789 604 Xanthe Terra
desc bottom-left</imagemap>
See also
References
- ^ Mars features itouchmap.com [dead link]
- ^ Irwin, R, et al. 2002. Geomorphology of Ma'adim Vallis, Mars and associated paleolake basins. J. Geophys. Res. 109(E12): doi:10.1029/2004JE002287
- ^ Michael H. Carr (2006). The surface of Mars. Cambridge University Press. ISBN 978-0-521-87201-0. Retrieved 21 March 2011.
- ^ "HiRISE | Light-toned Mounds in Gorgonum Basin (ESP_050948_1430)".
- ^ Blunck, J. 1982. Mars and its Satellites. Exposition Press. Smithtown, N.Y.
- ^ Osterloo; Hamilton, VE; Bandfield, JL; Glotch, TD; Baldridge, AM; Christensen, PR; Tornabene, LL; Anderson, FS; et al. (2008). "Chloride-Bearing Materials in the Southern Highlands of Mars" (PDF). Science. 319 (5870): 1651–1654. Bibcode:2008Sci...319.1651O. doi:10.1126/science.1150690. PMID 18356522. S2CID 27235249.
- ^ "NASA Mission Finds New Clues to Guide Search for Life on Mars". Jet Propulsion Laboratory. 2008-03-20. Retrieved 2008-03-22.
- ^ Murchie, S. et al. 2009. A synthesis of Martian aqueous mineralogy after 1 Mars year of observations from the Mars Reconnaissance Orbiter. Journal of Geophysical Research: 114.
- ^ Davila, A. et al. 2011. A large sedimentary basin in the Terra Sirenum region of the southern highlands of Mars. Icarus. 212: 579-589.
- ^ "HiRISE | Inverted Channels North of Juventae Chasma (PSP_006770_1760)". hirise.lpl.arizona.edu. Retrieved 6 September 2023.
- ^ "HiRISE | Gorgonum Chaos Mesas (PSP_004071_1425)". hirise.lpl.arizona.edu. Retrieved 6 September 2023.
- ^ "HiRISE | Gullies on Gorgonum Chaos Mesas (PSP_001948_1425)". hirise.lpl.arizona.edu. Retrieved 6 September 2023.
- ^ "HiRISE | Gullies in Newton Crater (PSP_004163_1375)". hirise.lpl.arizona.edu. Retrieved 6 September 2023.
- ^ U.S. department of the Interior U.S. Geological Survey, Topographic Map of the Eastern Region of Mars M 15M 0/270 2AT, 1991
- ^ "HiRISE | Spider Webs (ESP_046359_1250)". www.uahirise.org. Retrieved 6 September 2023.
- ^ Levy, J. et al. 2009. Concentric crater fill in Utopia Planitia: History and interaction between glacial "brain terrain" and periglacial processes. Icarus: 202. 462-476.
- ^ Levy, J., J. Head, D. Marchant. 2010. Concentric Crater fill in the northern mid-latitudes of Mars: Formation process and relationships to similar landforms of glacial origin. Icarus 2009, 390-404.
- ^ Levy, J., J. Head, J. Dickson, C. Fassett, G. Morgan, S. Schon. 2010. Identification of gully debris flow deposits in Protonilus Mensae, Mars: Characterization of a water-bearing, energetic gully-forming process. Earth Planet. Sci. Lett. 294, 368–377.
- ^ "HiRISE | Ice Deposition and Loss in an Impact Crater in Utopia Basin (ESP_032569_2225)". hirise.lpl.arizona.edu. Retrieved 6 September 2023.
- ^ Garvin, J., S. Sakimoto, J. Frawley. 2003. Craters on Mars: Geometric properties from gridded MOLA topography. In: Sixth International Conference on Mars. July 20–25, 2003, Pasadena, California. Abstract 3277.
- ^ Garvin, J. et al. 2002. Global geometric properties of martian impact craters. Lunar Planet. Sci: 33. Abstract # 1255.
- ^ "Catalog Page for PIA09662".
- ^ Kreslavsky, M. and J. Head. 2006. Modification of impact craters in the northern planes of Mars: Implications for the Amazonian climate history. Meteorit. Planet. Sci.: 41. 1633-1646
- ^ Madeleine, J. et al. 2007. Exploring the northern mid-latitude glaciation with a general circulation model. In: Seventh International Conference on Mars. Abstract 3096.
- ^ "HiRISE | Dissected Mantled Terrain (PSP_002917_2175)". hirise.lpl.arizona.edu. Retrieved 6 September 2023.
- ^ Fastook, J., J.Head. 2014. Concentric crater fill: Rates of glacial accumulation, infilling and deglaciation in the Amazonian and Noachian of Mars. 45th Lunar and Planetary Science Conference (2014) 1227.pdf
- ^ Barlow, N. 2008. Mars: An Introduction to its Interior, Surface and Atmosphere. Cambridge University Press
- ^ Forget, François; Costard, François; Lognonné, Philippe (12 December 2007). Planet Mars: Story of Another World. Praxis. ISBN 978-0-387-48925-4.
- ^ Taylor, Fredric W. (10 December 2009). The Scientific Exploration of Mars. Cambridge University Press. ISBN 978-0-521-82956-4.
- ^ Connerney, J. et al. 1999. Magnetic lineations in the ancient crust of Mars. Science: 284. 794-798.
- ^ Langlais, B. et al. 2004. Crustal magnetic field of Mars. Journal of Geophysical Research. 109: EO2008
- ^ Connerney, J.; Acuña, MH; Ness, NF; Kletetschka, G; Mitchell, DL; Lin, RP; Reme, H; et al. (2005). "Tectonic implications of Mars crustal magnetism". Proceedings of the National Academy of Sciences of the USA. 102 (42): 14970–14975. Bibcode:2005PNAS..10214970C. doi:10.1073/pnas.0507469102. PMC 1250232. PMID 16217034.
- ^ Acuna, M.; Connerney, JE; Ness, NF; Lin, RP; Mitchell, D; Carlson, CW; McFadden, J; Anderson, KA; et al. (1999). "Global distribution of crustal magnetization discovered by the Mars Global Surveyor MAG/ER Experiment". Science. 284 (5415): 790–793. Bibcode:1999Sci...284..790A. doi:10.1126/science.284.5415.790. PMID 10221908.
- ^ "ESA Science & Technology - Martian Interior". sci.esa.int. Retrieved 6 September 2023.
Recommended reading
- Grotzinger, J. and R. Milliken (eds.). 2012. Sedimentary Geology of Mars. SEPM.
- Lorenz, R. 2014. The Dune Whisperers. The Planetary Report: 34, 1, 8-14
- Lorenz, R., J. Zimbelman. 2014. Dune Worlds: How Windblown Sand Shapes Planetary Landscapes. Springer Praxis Books / Geophysical Sciences.
External links
Lua error in mw.title.lua at line 346: bad argument #2 to 'title.new' (unrecognized namespace name 'Portal').